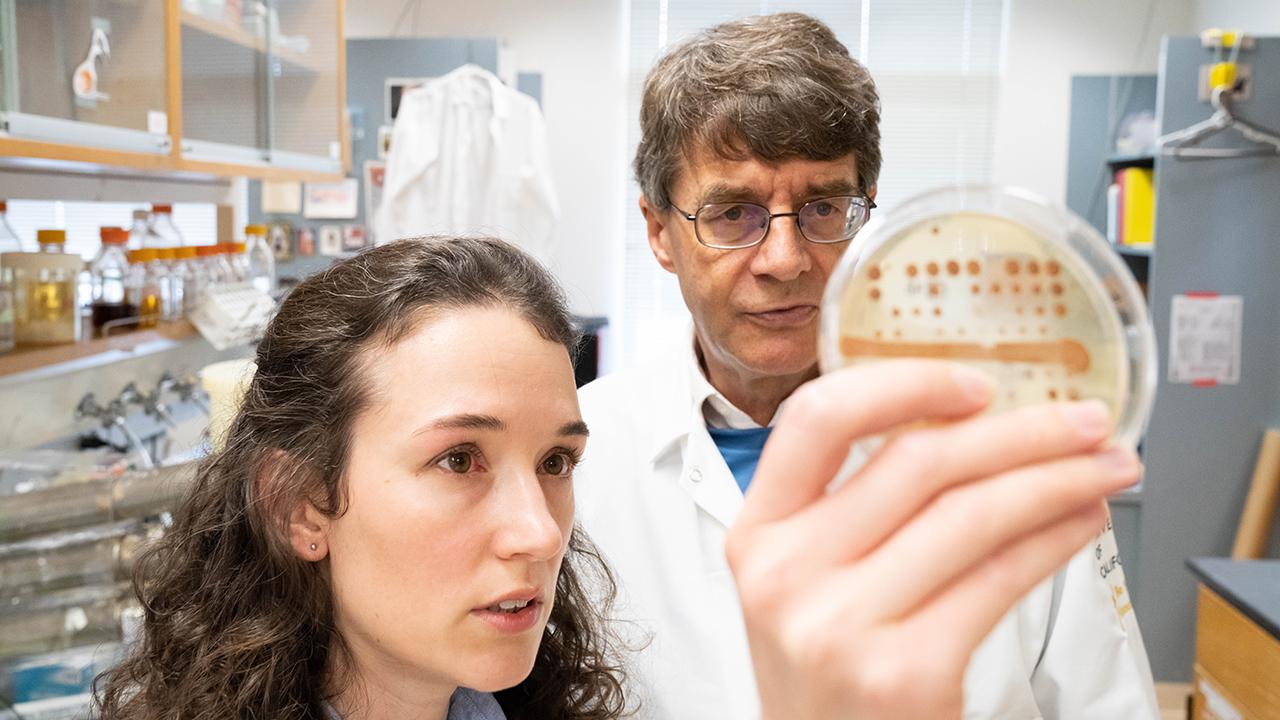
Experiments in Yeast Hint at Possible Origins of Cancer and Autism
New study reveals mechanisms of genomic instability
Cancer often starts with the reshuffling of DNA—akin to scrambling the pages of a dictionary. Exactly how this happens has long been a mystery. But researchers in the UC Davis College of Biological Sciences have now arrived at one promising explanation.
The problem seems to happen at a critical moment: when the cell is fixing a broken string of DNA. This repair process, called homologous recombination, can go awry, says Wolf-Dietrich Heyer, a Distinguished Professor and chair in the Department of Microbiology and Molecular Genetics.
“It’s counterintuitive,” he says. “A mechanism for maintaining genome stability can actually cause instability.”
Heyer and his students have studied this process for years. Their latest discovery, published August 4 in the journal Genes & Development, could shed light on the origins of cancer, and of neuro-developmental disorders such as autism.
Mismatched DNA
Homologous recombination depends on the fact that human cells contain two complete copies of the genome, divided into 23 pairs of matching chromosomes (and when a cell has duplicated its DNA in preparation for dividing, it briefly has four copies of its genome). When the DNA on one chromosome is broken—a so-called double-stranded break—the homologous DNA sequence from a matching chromosome can be used as a template to repair the DNA break.
It’s a difficult task, because before the cell can repair its DNA, its proteins must first find the matching sequence—the equivalent of finding “one particular piece of hay in a haystack,” says Heyer.
The problem is that some stretches of DNA have more than one match. This is where the repair process sometimes goes astray—triggering a genomic catastrophe.
The genomes of humans and all other plants, animals and fungi are full of what some researchers call “junk” DNA sequences, which are repeated and scattered thousands of times across the genome. If some of these repeats happen to sit near the broken end of the DNA molecule, then the cell’s proteins might erroneously match the broken DNA to more than one chromosome.
“Instead of using a single homologous chromosome, the cell ends up using multiple regions of the genome to repair the broken DNA,” says Diedre Reitz, the postdoctoral fellow in Heyer’s lab, who is lead author on the new study.
When multiple matches are made, the cell’s DNA, which is normally a linear molecule similar to a ladder, ends up in a tangle with branches in multiple places. As the cell’s proteins unwind that tangle, they clip the DNA in the wrong places, introducing numerous errors.
As a result, the DNA thread that was broken ends up spliced onto a sequence that it wasn’t previously connected to—creating an error called a “translocation.” At this point, the formerly intact DNA strands that were used as repair templates are now broken off—requiring another round of repair—with the potential for compounding errors.
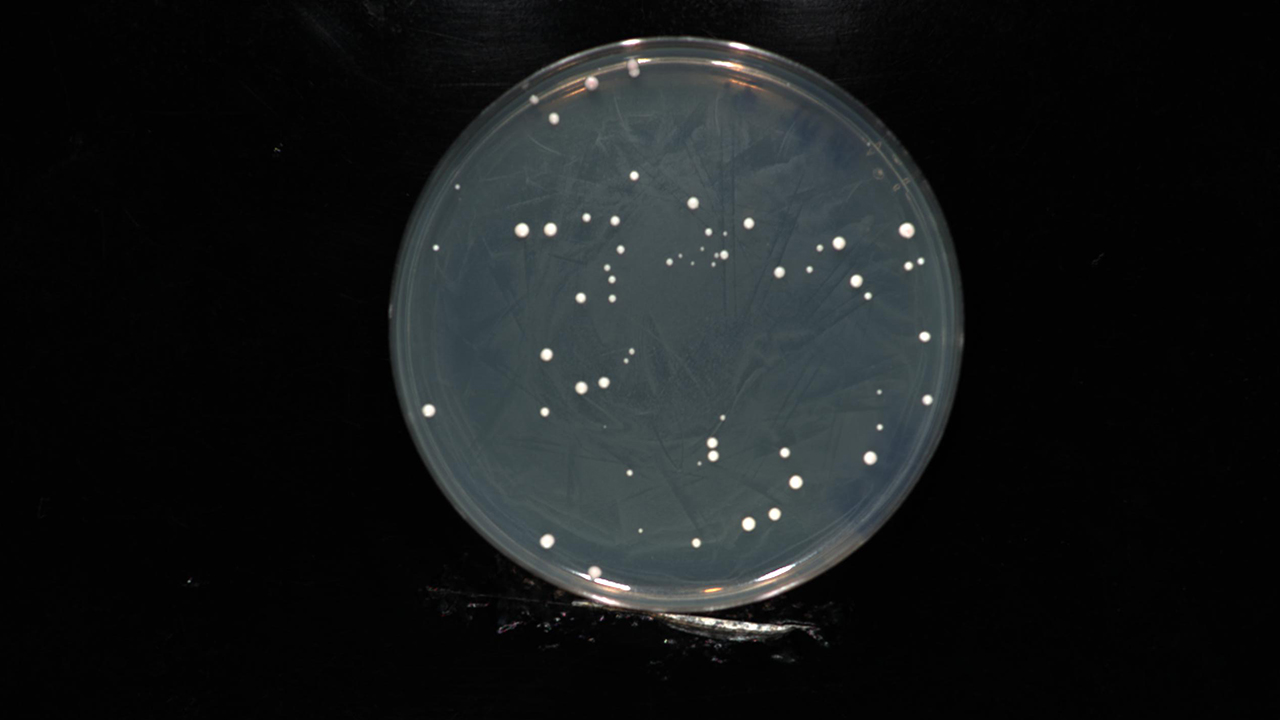
“This process can generate a cascade of re-arrangements,” says Heyer, as one botched repair leaves newly broken DNA threads—which themselves are repaired incorrectly—producing yet more broken threads.
The newly published paper shows that these DNA breaks and incorrect repairs happen in Saccharomyces cerevisiae yeast, an established model in genomics research. Given the evolutionary similarities of the DNA repair proteins between yeast and animals, this may also happen in human disease.
“This signature has been observed in many cancers, and in people with certain developmental problems,” says Heyer. “They have what are essentially shattered genomes, in which the DNA of affected cells has dozens or even hundreds of individual breaks and re-arrangements.”
This genome shuffling plays a fundamental role in generating cancer cells. So-called tumor suppressor genes, which prevent uncontrolled cell division, can be lost. And “oncogenes,” which promote rapid cell division, can be turned on at full blast.
Surprising Pathways
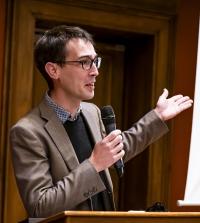
This new research builds on an experimental yeast model that was first published in 2017 by Aurèle Piazza, while he was a post-doctoral fellow in Heyer’s lab at UC Davis.
Piazza, who is now a research team leader at the Ecole Normale Supérieure in Lyon, France, also played a pivotal role in the new research. He further adapted his experimental system, using a DNA method called polymerase chain reaction (PCR) to detect DNA repair errors that are vanishingly rare.
The new experiments revealed that these errors in DNA repair can happen through two distinct pathways. One pathway, which was expected, requires the cell to synthesize new DNA, says Piazza. “But you can also get DNA re-arrangements that are massive, but independent of DNA synthesis,” he says—and this was a surprise.
It’s an important discovery because DNA re-arrangements, and cancer, often arise in cells that don’t normally have the capacity to divide, or synthesize new DNA. The discovery could also be important for understanding autism and other neurodevelopmental diseases: patients with these conditions are sometimes found to harbor DNA re-arrangements in their nerve cells—which also tend not to synthesize DNA. In fact, these neural disorders can involve mutations in some of the same genes as cancer.
The newly identified pathways generate specific fingerprints in the DNA sequence. Reitz, Heyer, and their colleagues are starting to look for those fingerprints in tumor samples from cancer patients. Finding them would provide evidence that what they discovered in yeast really could be an important trigger for human disease – an insight which may one day even affect treatment decisions.
Media Resources
- Douglas Fox is a freelance science writer based in the Bay Area.
- Delineation of two multi-invasion-induced rearrangement pathways that differently affect genome stability (Genes & Development 2023)
- Multi-invasions Are Recombination Byproducts that Induce Chromosomal Rearrangements (Cell 2017)
- Autism and Cancer Share Risk Genes, Pathways, and Drug Targets (Trends in Genetics 2016)
- Heyer Lab