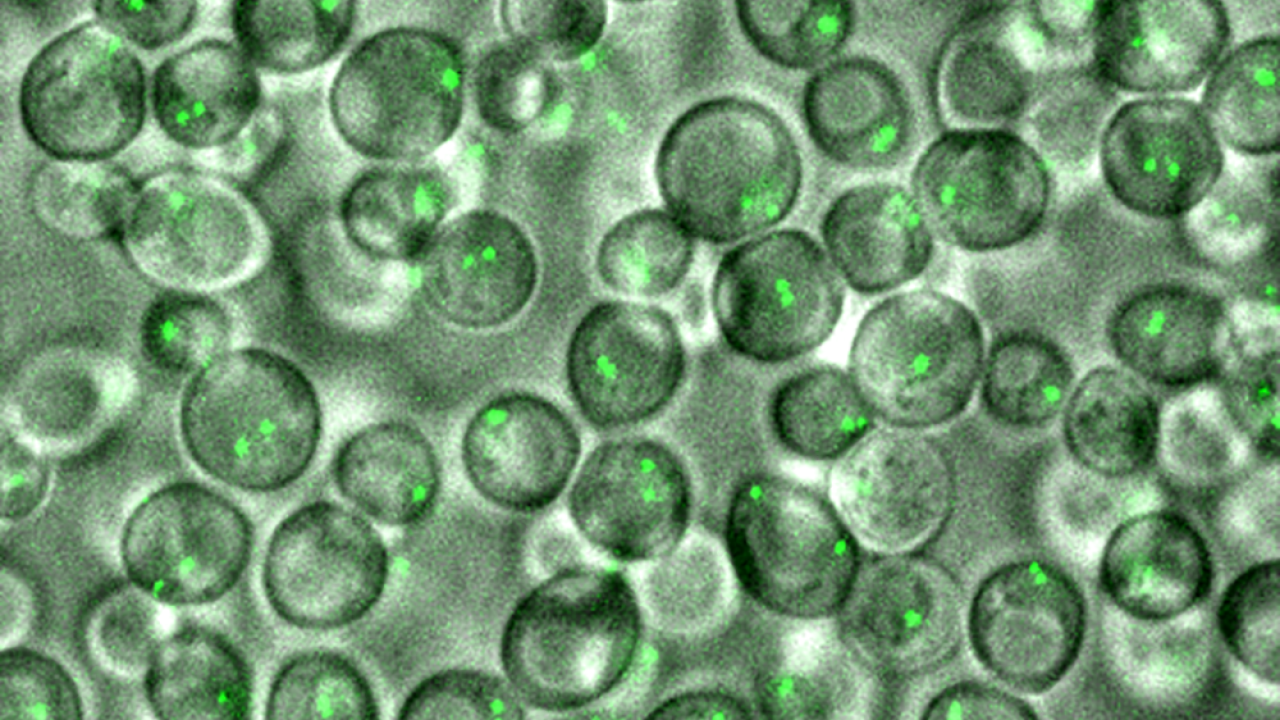
Tenuous Tethers: Study Provides Live View into Interchromosomal Dynamics During Meiosis
In the choreography of meiosis—the process responsible for sex cell division in all eukaryotic life—the pairing of homologous chromosomes (homologs) is essential. Errors in this process can lead to an incorrect number of chromosomes in sex cells, which can result in birth defects and miscarriages. Despite being studied for more than 100 years, mysteries about the process still abound.
“Homolog pairing has been seen for 130 years, but the mechanism behind it is not really well understood,” said Professor Sean Burgess, Department of Molecular and Cellular Biology. “Most studies looking at chromosomes in meiosis focus on cells that have been fixed, so it’s just a snapshot that shows whether the homologs are paired or not.”
In a study appearing in the Proceedings of the National Academy of Sciences, Burgess and her colleagues provide an unprecedented glimpse into the homolog pairing phase of meiosis (prophase I). Using yeast (Saccharomyces cerevisiae) as a model organism, the team successfully observed homolog pairing in real-time.
“What we found was really surprising,” said Burgess. “We expected that the chromosomes would start out unpaired and then over time, they would get closer and closer together and progressively more and more paired.”
Instead, the team uncovered that the chromosomes weren’t stable during homolog pairing. Their movements were dynamic, with only a few tethers required to keep a pair bound together. The study provides the first live view into interchromosomal dynamics during meiosis.
“We know that birth defects and miscarriages can arise from defects that occur in meiosis prophase that are related to pairing and recombination,” said Burgess. “So understanding the basic biology helps us understand how these errors can arise.”
A few strong links
During meiosis, hundreds of double-strand breaks (DSBs) occur on a chromosome. This process is necessary for homolog pairing, with the breaks creating crossovers that physically link two homologs together. In budding yeast, homolog pairing depends on roughly 150 to 200 DSBs that are mediated by the gene Spo11.
To get a glimpse at the process, the team created a strain of budding yeast that possessed chromosomes with fluorescent markers. Using light imaging microscopes, they then watched how those sites behaved during the entirety of the meiosis prophase, taking measurements every 30 seconds over 5 or 6 sequential 25-minute periods. An algorithm Burgess’ lab developed SeeSpotRun enabled them to determine the position of these chromosomal sites in 3D space.
“What we found was that the process was very dynamic, much more than we expected,” said Burgess. “The chromosomes were moving all the way through meiotic prophase…They weren’t necessarily becoming stable pairs, so this was kind of confusing to us.”
A union of biology and physics
To make sense of the finding, Burgess reached out to Professor Andrew Spakowitz and Bruno Beltran, both of Stanford University. Using parameters defined by Burgess that replicate the environment of the nucleus, the Stanford team successfully modeled how homologous pairs behave during the various stages of meiotic prophase. What they saw in their model matched the data Burgess’ team collected during their observations of homolog pairing in yeast.
“Using their polymer theory, they determined that if you had two polymers randomly diffusing and you put random linkages between them, they could model how the chromosomes would move,” said Burgess. “The linkages in the polymer model were the same number of special crossovers that are normally found during meiosis.”
“So even though there are a lot of DSBs, you only end up with a few of these crossovers,” she added, noting that only between two and four linkages were required to keep homolog pairs together. It is known from other work that the formation of these linkages creates an inhibitory signal that prevents other linkages from forming nearby. This could explain why only two to four linkages are found per homolog pair.
Burgess will continue working with her Stanford colleagues to further probe the homolog pairing process. “We want to look at the dynamics of the pairing in mutants that disrupt those special recombination sites to see if the linkages are in fact due to those special recombination events,” she said.
The research will help further illustrate the processes behind homolog pairing and shed light on longstanding questions, including what enables successful homolog pairing and how to prevent errors.
The research is funded by grants from the National Institutes of General Medicine and the National Science Foundation. Postdoctoral fellow Trent Newman is first author on the publication.
Media Resources
- Read the paper in PNAS